Retinal ischemia
The retina has a high metabolic demand and therefore reacts very sensitively to reduced blood flow (ischemia). Ischemic processes therefore play an important role in the pathophysiology of various eye diseases. These include glaucoma, ocular vascular occlusions and diabetic retinopathy. The therapeutic options are currently limited and require analysis.
Ischemia in the retina leads to an oxygen deficiency in the tissue, which leads to cell death and functional and morphological changes. When the tissue subsequently becomes perfused again (reperfusion), the increased oxygen and the associated aggressive oxygen metabolites also have toxic effects on the neuronal cells.
Ischemia/reperfusion model (I/R model)
Using the I/R model, our working group investigates the impact of ischemic processes on the retina and optic nerve and tries to decipher the molecular mechanisms behind them.
To do this, we analyzed the influence of ischemic processes over time (2, 6, 12 and 24 hours, 3 and 7 days). We were able to show that there is a significant loss of retinal ganglion cells as early as 2 hours after I/R. The damage increases progressively over time and manifests itself in a reduction in retinal layer thickness and in the loss of neuronal cells (Figure 1; Palmhof et al. 2019). Retinal ganglion cells in particular are affected, but also amacrine cells, bipolar cells and photoreceptors (Schmid et al. 2014, Palmhof et al. 2019). In addition, due to the severe tissue damage, the number of inflammatory microglia cells increases and there is a loss of function of the inner retinal cell layers (Palmhof et al. 2019, Wagner et al. 2020).
Based on our analyses of ischemic optic nerves, we were able to show that severe tissue damage also occurs there in the form of cell infiltration, demyelination and immigration and activation of immune effector cells (Palmhof et al. 2020).
Investigations into the underlying cell death mechanisms showed that apoptosis (Schmid et al. 2014, Wagner et al. 2021) and autophagy (Palmhof et al. 2018) are involved.
Figure 1: Time course of retinal ganglion cell loss after ischemia.
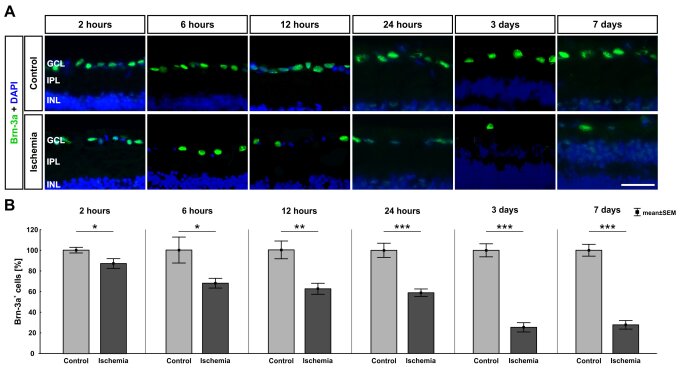
(A) Retinal ganglion cells were visualized with Brn-3a (green), cell nuclei with DAPI (blue). (B) A significant loss of retinal ganglion cells was observed as early as 2 hours after ischemia (p=0.032). This loss increased over time (6 hours: p=0.026, 12 hours: p=0.002, 24 hours, 3 and 7 days: p<0.001). Abbreviations: GCL=ganglion cell layer, IPL=inner plexiform layer, INL=inner granular layer. Scale bar: 20 μm; B: n=7-8/group, C: n=5/group; *: p<0.05; **: p<0.01; ***: p<0.001 (Palmhof et al. 2019).